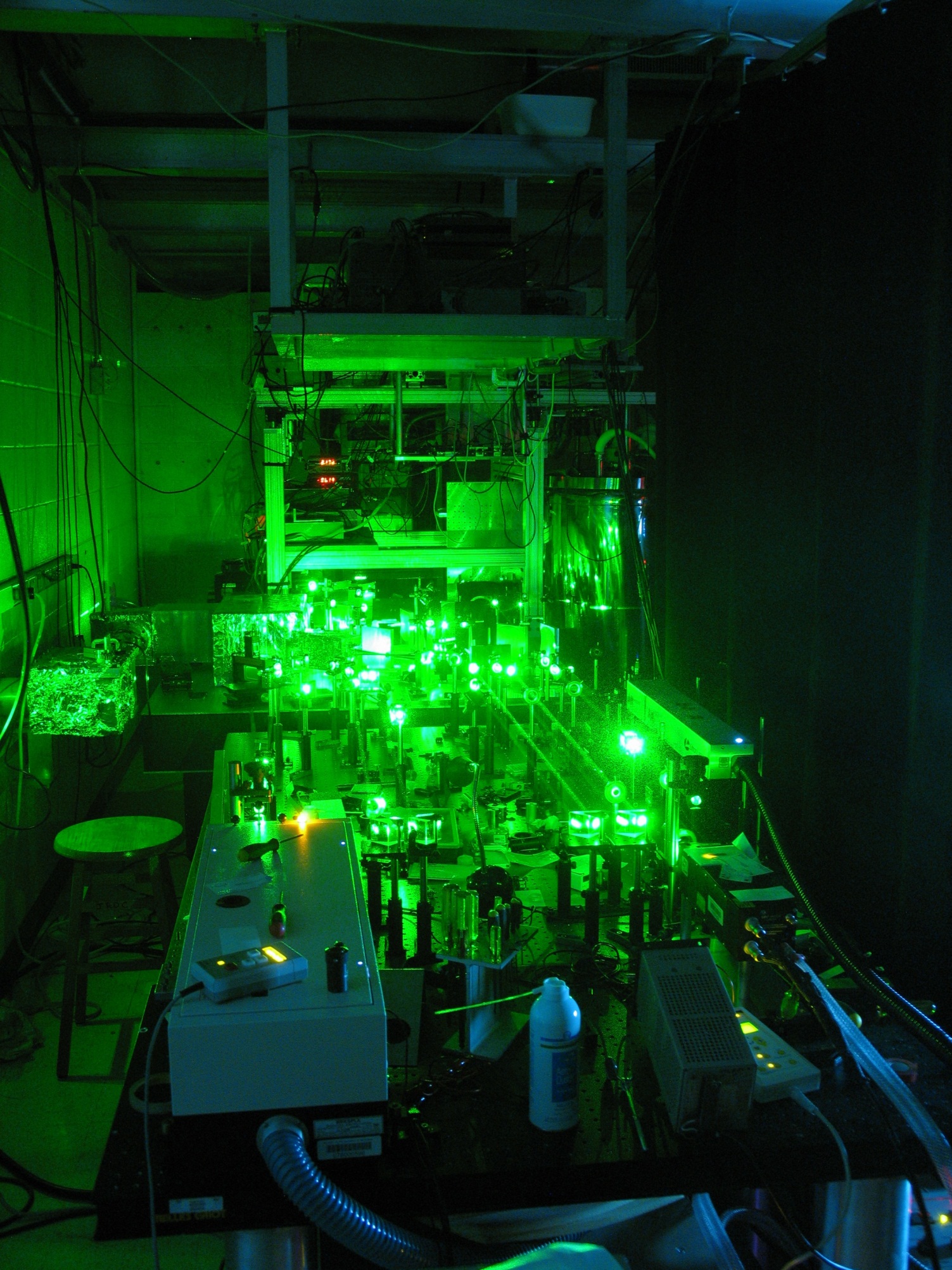
Figure 1: Nd:YAG lasers. High-power green lasers are used to drive the switches that generate up to two pulses from the FEL. This makes the lab bright during operation (and lasers goggles a must)!
Electron Paramagnetic Resonance (EPR) is a sensitive probe of the local environment around a paramagnetic species. EPR can be used to study a variety of systems in physics, material science, chemistry, and biology. In particular, EPR has proven to be exceptionally important in molecular biology where ‘tagging’ a biomolecule with a stable paramagnetic allows site-specific measurements of structure and function of proteins and other biological systems. EPR becomes more powerful as the applied magnetic field is increased, but applications of high-field EPR are limited by to the absence of suitable high-power sources. In the Sherwin lab we are developing novel EPR instrumentation by using the UCSB Free Electron Lasers (FELs) to do high-powered, pulsed EPR at fields more than twice as large as previously possible. The UCSB FELs offer ~kWatts from 100 GHz to 5 THz, a range of frequencies where typically less than 1 Watt is available. Currently operating at 240 GHz, special switches driven by powerful, green lasers (see fig. 1) are used to generate up to 2 pulses with significant tuning of lengths and separation to excite spins faster. The FELEPR project has led to the operation of the highest-field, high-power pulsed EPR spectrometer as well as offering a platform for instrument and method development for high-field EPR.
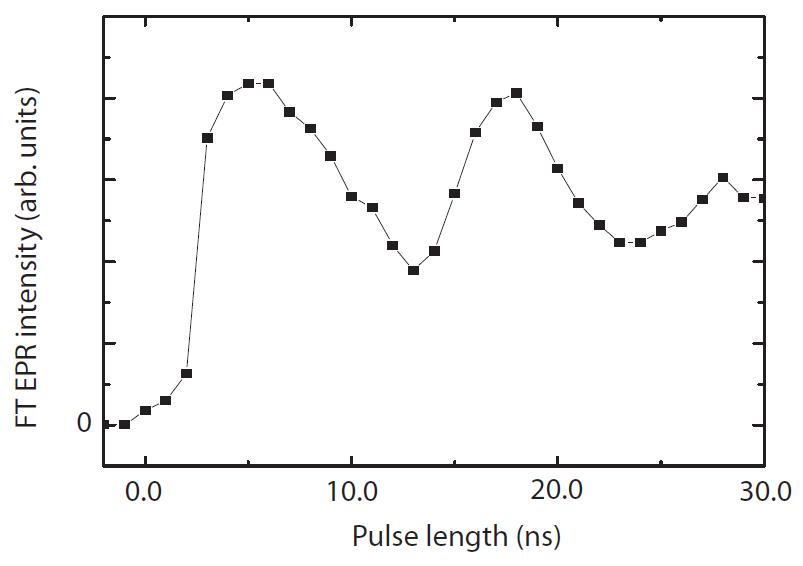
Figure 2: Rabi oscillations. The Rabi oscillations demonstrate our ability to excite spins in only 6 ns (by tipping them 90°). This is only possible due to the high power produced by the FEL, and is ~50-100 times faster than with other sources.
As a result of the extremely high power offered by the FELs, the capabilities of the FELEPR spectrometer are transformative compared to other, low-power sources operating at similar fields. By measuring the pulse length necessary to rotate the spins (Rabi oscillations), we can characterize the strength of the pulses we are applying. The measured Rabi oscillations are shown in fig. 2 and demonstrate a ~13 ns 180º pulse (from the first maximum, to the second), corresponding to a 90-degree length of ~6.5 ns, roughly 50-100 times faster than possible with other spectrometers at similar frequencies. A further advantage of the short pulses used here is the substantial increase in excitation bandwidth achieved. This is demonstrated in Fig.3, where the EPR signal from a nitrogen impurity in diamond (split into 3 lines by the coupling to the S=1 nitrogen nucleus) is shown with all three lines being excited. Using a longer pulse, as is necessary at lower powers, the narrow excitation bandwidth prevents observing more than one line at a time. Finally, we also carry out 2-pulse echo measurements, and FELEPR can be shown to measure relaxation times ten times faster than is possible with low power sources, as is shown in fig. 4, where the echo is detected within ~300 ns, half of the typical length of a single pulse on other spectrometers. The FELEPR spectrometer, while operational, is regularly being upgraded. Ongoing work includes on recently implemented phase cycling techniques, and work towards measurements of the electron T1e.
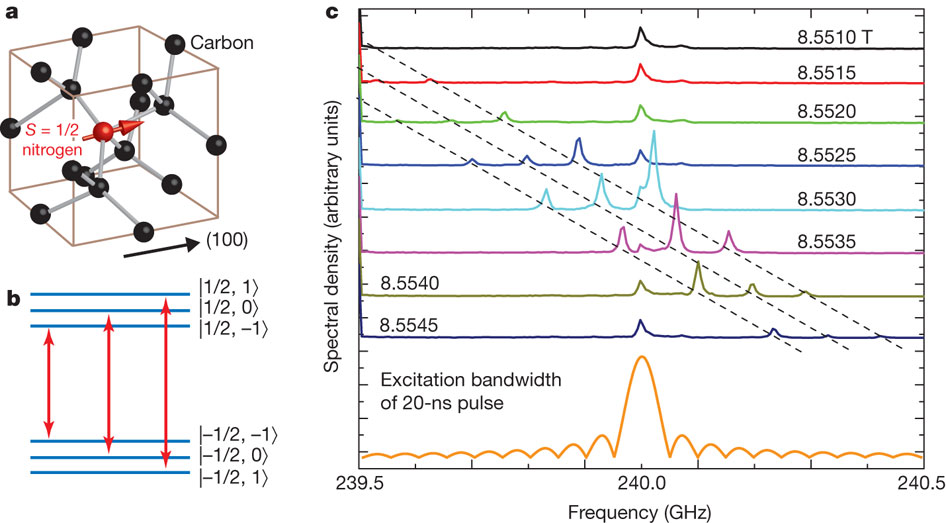
Figure 3: Extended excitation bandwidth. By utilizing short pulses we dramatically expand the excitation bandwidth of our pulses. This improves sensitivity and allows probing multiple populations at once, as is shown for the three hyperfine lines in a nitrogen vacancy in diamond.
UCSB Researchers:
- PIs:
- Mark Sherwin
- Songi Han (Chemistry and Biochemistry)
- Graduate Students:
- Blake Wilson (Physics)
Collaborators:
Publications:
- D.T. Edwards, Y. Zhang, S.J. Glaser, S. Han, M.S. Sherwin, Phase Cycling with a 240 GHz, Free Electron Laser-powered Electron Paramagnetic Resonance Spectrometer, Physical Chemistry Chemical Physics, 15, 5707-5719 (2013) [www]
- S. Takahashi, L.-C. Brunel, D.T. Edwards, J. van Tol, G. Ramian, S. Han, M.S. Sherwin, Free-Electron Laser-Powered Electron Paramagnetic Resonance Spectroscopy, Nature, 489, 409–413 (2012) [www]
- S. Takahashi, G. Ramian, M.S. Sherwin, Cavity dumping of an injection-locked free-electron laser, Appl. Phys. Lett. 95, 234102 (2009) [www]
- S. Takahashi, D.G. Allen, J. Seifter, G. Ramian, M.S. Sherwin, L.-C. Brunel, J. van Tol, Pulsed EPR spectrometer with injection-locked UCSB free-electron laser, Infrared Physics & Technology, 51, 426-428 (2008) [www]
- S. Takahashi, G. Ramian, M.S. Sherwin, L.-C. Brunel, J.v. Tol, Submegahertz linewidth at 240 GHz from an injection-locked free-electron laser, Appl. Phys. Lett., 91, 174102 (2007) [www]