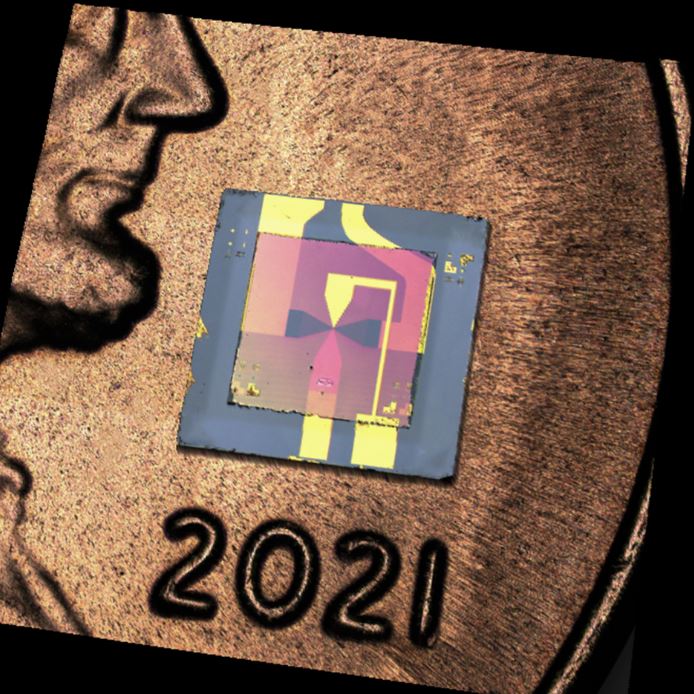
In this project, we are developing a near-quantum-noise limited THz heterodyne sensor for high-resolution spectroscopy in planetary and cometary missions. Currently, all near-quantum-limited THz heterodyne mixers for astrophysics applications use superconductors and hence require active cryogens or mechanical coolers to operate at near 4 K. On the other hand, only much noisier Schottky diode mixers, which can operate at ~ 100 K, have been available for planetary and cometary applications. Named as Tunable-Antenna Coupled Intersubband Terahertz (TACIT) mixer, our detector aims to enjoy the best of both worlds by offering near quantum-limited noise performance at relatively high operating temperatures (50 – 70 K) which are accessible with passive cooling in deep space. In addition, TACIT mixers can have in-situ tunability in the detection frequency (2 – 5 THz) via small (< ~3V) gate biases and are expected to require relatively low required pump power (~ 1 μW). These advantages of TACIT mixers will enable sensitive and tunable THz heterodyne instruments that can operate on passive cooling in multi-pixel configuration that will be attractive for high-resolution THz heterodyne spectroscopy in future planetary and cometary applications.
TACIT mixer is a four-terminal device based on a high-mobility 2-dimensional electron gas (2DEG) confined in a GaAs/AlGaAs quantum well that uses intersubband transitions for efficient absorption of THz radiation (Fig. 1(a)). Two of the four terminals are ohmic contacts to the 2DEG (source and drain) that are used to DC-bias the source-drain channel and to read out the device response in the in-plane resistance of the 2DEG. The other two terminals are top and bottom gates sandwiching the quantum well in the active region where THz absorption occurs. The THz absorption frequency is primarily determined by the width of the quantum well (for example, we use a 40-nm quantum well to center our absorption frequency near 2.4 THz) and can be further tuned in-situ by varying the charge density in the quantum well and the DC electric field applied along the growth direction of the quantum well. The top and the bottom gates are used to apply additional DC biases to independently tune the charge density and the DC electric field to match the absorption frequency of the device to incoming THz radiations. Once the THz absorption occurs, the absorbed THz energy quickly raises the electron temperature of the 2DEG and hence the in-plane resistance of the device through the strong non-linear dependence of the mobility of a high-mobility 2DEG on electron temperature. When a THz local oscillator (LO) signal is provided with the THz signal (referred as RF) to the device, this fast bolometric response in the in-plane resistance of the device can follow the difference frequency between the THz RF and LO (the intermediate-frequency signal) that usually lies in the microwave region.
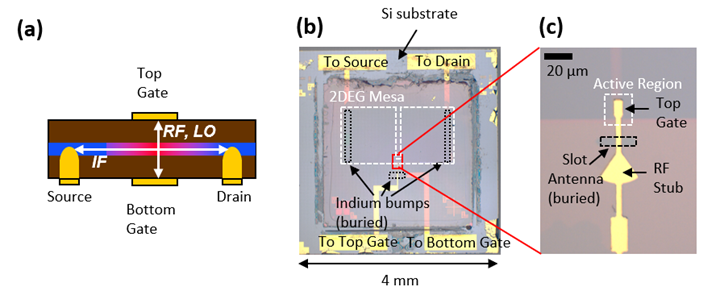
We recently fabricated and characterized a prototype TACIT mixer based on quasi-optical coupling scheme (see Figs 1(b) and 1(c)). In this coupling method, the prototype TACIT mixer was mounted on a Si lens that focuses incoming THz radiation into the active region of the device. In the active region of the device, a single slot antenna integrated with the top and the bottom gates further couples the focused THz radiations into the quantum well (Fig 1(c)). Figures 3 – 5 show the characterization results of the prototype device demonstrating the gate tunability in the current-voltage (IV) characteristics of the device (Fig. 3), the tunability in the direct detection response (Fig. 4), as well as the THz heterodyne detection (Fig. 5).
Currently, we are at the most exciting phase of the development for TACIT mixer technology where a lot of important work can be done to improve and better understand TACIT mixers. First, we are further developing quasi-optically-coupled TACIT mixers with improved THz antenna design and device geometry. These devices are expected to have better THz and IF coupling efficiencies and will be used for sensitivity characterization of TACIT mixers. In addition, we plan to implement waveguide coupling in our device to further enhance the THz coupling efficiency for better sensitivity and, more importantly, to enable multi-pixel application of TACIT mixer technology.
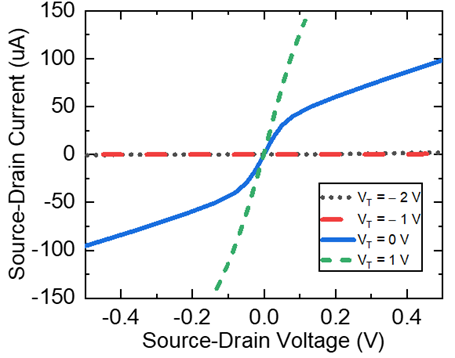
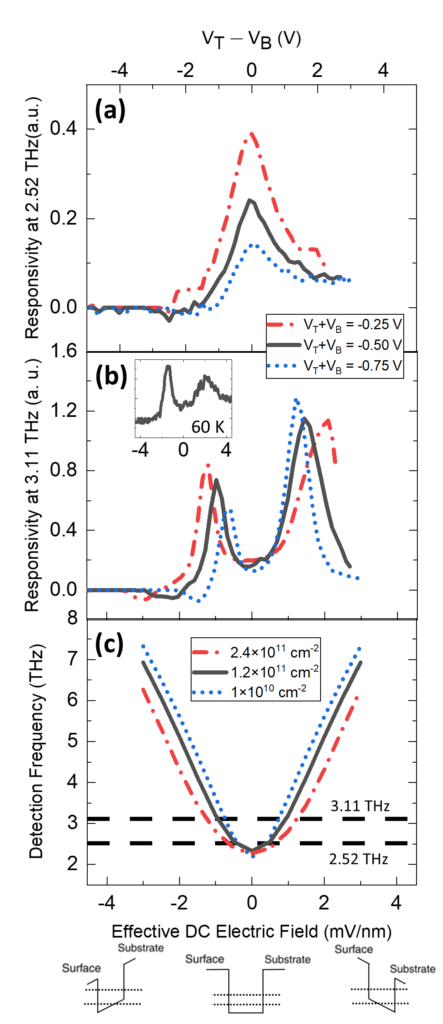
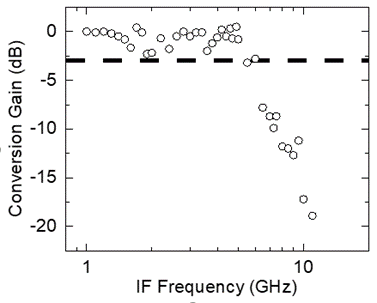
UCSB Principal Investigator:
Prof. Mark Sherwin (Physics)
Graduate Student:
Changyun Yoo (Physics)
Collaborators:
Dr. Boris Karasik (JPL, PI)
Dr. Jonathan Kawamura (JPL)
Dr. Kenneth West (Princeton University)
Dr. Loren Pfeiffer (Princeton University)
Publication:
Demonstration of a Tunable Antenna-Coupled Intersubband Terahertz (TACIT) Mixer
Changyun Yoo, Mengchen Huang, Jonathan Kawamura, Kenneth West, Loren Pfeiffer, Boris Karasik, Mark Sherwin
Applied Physics Letters 116 013504 (2020) [www]