Intense laser fields can rip electrons from an atom and slam them back into the ionic core it left behind. By using intense terahertz radiation, we have extended this idea to electrons and holes in solids, driving the two away from each other and recolliding them at higher energies. High-order sideband generation (HSG) is a highly nonlinear optical process. HSG involves two frequencies of light: relatively weak near-infrared (NIR) light and very intense THz light. When the two are combined in a high-mobility semiconductor material, many new frequencies are created, as can be seen in Fig. 1. These new frequencies, or sidebands, are around the initial NIR laser frequency with a spacing of twice the THz frequency, or fSB = fNIR + 2 n*fTHz. HSG can be understood semiclassically using a three-step model, shown as a cartoon in Fig. 2. First, a bound electron-hole pair is created by the NIR laser tuned to the band gap of the material. Simultaneously, the very intense THz electric field created by the UCSB FEL warps the Coulomb potential felt by the electron so much that the electron tunnels away from the hole. Second, the electron and hole are free to accelerate in the strong THz field, first away from and then back towards each other. Third, the particles recollide with one another with significant kinetic energy. When the particles recollide, they annihilate and emit a photon with more energy than the original NIR photon, creating the sidebands.
Initial HSG experiments conducted with a photomultiplier tube (PMT), first reported in Nature in 2012, observed 9 sidebands in InGaAs/AlGaAs quantum wells. Subsequent HSG experiments observed 11 sidebands in GaAs/AlGaAs (PRL 2013) and 8 sidebands in bulk GaAs, 5 of which persisted to 170 K (APL 2013). An optics upgrade which incorporated a CCD into the sideband detection scheme greatly increased the ability to observe sidebands. The most recent HSG papers have reported the observation of 45 and 55 sidebands (PRX and Optics Express), to our knowledge the highest-order optical nonlinearity reported in solids.
Beyond observing high-order optical nonlinearities from a variety of semiconductor systems, recent HSG experiments expanded the amount of information gained from sidebands. The sideband polarization states contain information about the effective Hamiltonian that governs the electron-hole pairs and is determined by the quantum interference of different electrons and holes that produce the same sideband (Fig. 2). Recent experiments allowed for an all optical reconstruction of the Bloch wavefunctions of the hole states in GaAs, shown in Fig. 3. More than 90 years after the proof of Bloch’s theorem, this is, to our knowledge, the first time Bloch wavefunctions have been reconstructed directly from angle-resolved experimental data. Further investigations of sideband polarization offer exciting research opportunities, with the goal of reconstructing the properties of wavefunctions in condensed matter systems. HSG experiments on exotic systems remain an enticing possibility, especially for measuring and parameterizing topological quantities of interest like the Berry phase and Berry curvature.
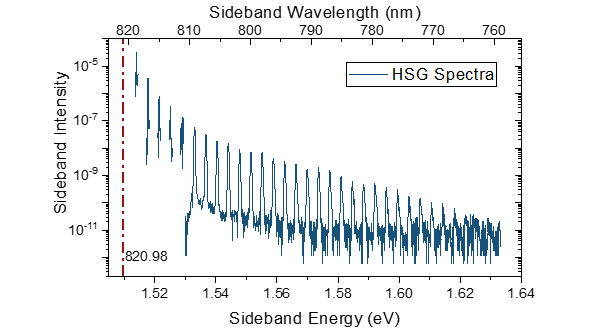
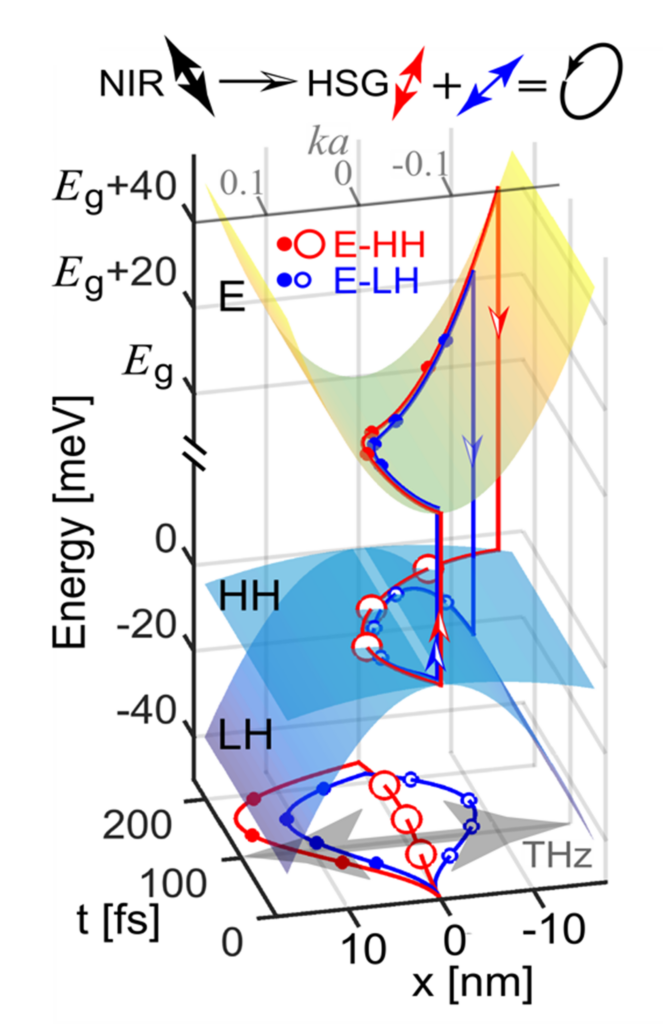
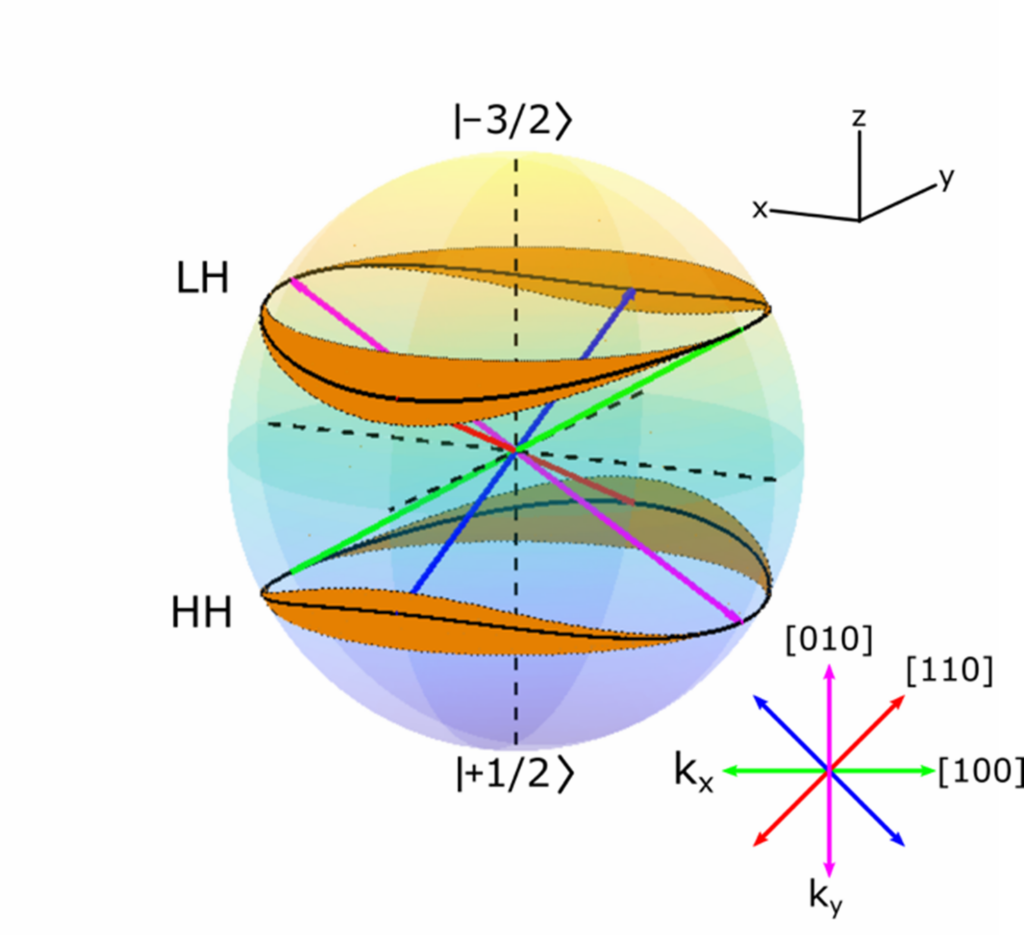
Principal Investigator:
Prof. Mark Sherwin (Physics)
Graduate Students:
Joe Costello (Physics)
Seamus O’Hara (Physics)
Optical Frequency Combs from High-Order Sideband Generation
Valovcin, Darren; Banks, Hunter B.; Mack, Shawn; Gossard, Art; West, Ken; Pfeiffer, Loren; Sherwin, Mark S.
Optics Express 26, 29807-29816 (2018) [www]
Dynamical Birefringence: Electron-Hole Recollisions as Probes of Berry Curvature
Banks, Hunter B.; Wu, Qile; Valovcin, Darren C.; Mack, Shawn; Gossard, Arthur C.; Pfeiffer, Loren; Liu, Ren-Bao; Sherwin, Mark S.
Physical Review X 7, 041042 (2017) [www]
Lightwave-Driven Quasiparticle Collisions On A Subcycle Timescale
Langer, F.; Hohenleutner, M.; Schmid, C. P.; Poellmann, C.; Nagler, P.; Korn, T.; Schueller, C.; Sherwin, M. S.; Huttner, U.; Steiner, J. T.; Koch, S. W.; Kira, M.; Huber, R.
Nature 533, 225 (2016) [www]
Near Infrared Frequency Dependence Of High-Order Sideband Generation
Zaks, Benjamin; Banks, Hunter; Liu, Ren-Bao; Sherwin, Mark
Physics Of Semiconductors 1566, 429 (2013) [www]
Terahertz Electron-Hole Recollisions In Gaas/Algaas Quantum Wells: Robustness To Scattering By Optical Phonons And Thermal Fluctuations
Banks, Hunter; Zaks, Ben; Yang, Fan; Mack, Shawn; Gossard, Arthur C.; Liu, Renbao; Sherwin, Mark S.
Physical Review Letters 111, (2013) [www]