New Single Shot Frequency Monitor
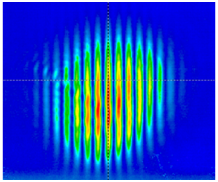
Semiconductor Processing
UCSB is a world leader in III-V semiconductor processing and molecular beam epitaxy (MBE). The state-of-the-art Nanofabrication Facility, one of the premier university cleanroom facilities in the country, was recently augmented by the engineering sciences cleanroom–occupying the entire first floor of a brand new 90,000 sq. ft. building.Terahertz Sources – all in one convenient location
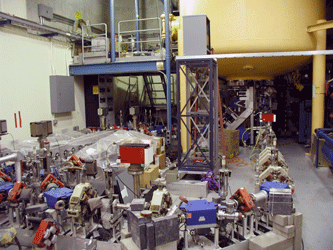
CW Virginia Diodes coherent sources: 240 GHz 1THz. These sources made by Virginia Diodes multiply a 13 GHz coherent microwave source up to 240 GHz in one source and 1 THz in the second source. These sources are tunable around these central frequencies.
Terahertz Spectrometers
- THz time domain systems:
- ASOPS: THz-TDS fiber laser based system with no mechanical delay line, capable of 500ns/spectrum
- Traditional THz-TDS system
- Bruker FTIR spectrometer spectrometer (Michelson interferometer w/ coherent broadband source)
- 240 GHz pulsed ESR spectrometer
Terahertz Optics – unique expertise
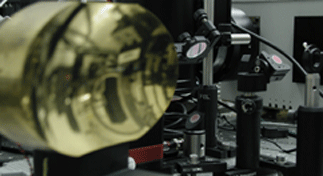
- Wire-grid polarizers
- Variable retardation plates
- Large off-axis parabolic mirrors and elliptical mirrors
- TPX THz lenses
Optical sources, detectors
- Doubled Mode-locked Ti:S laser ([350-400nm], 700-800nm, 150 fs pulses)
- Regenerative Ti:S amplifier (10 mJ per pulse)
- CW Tunable Ti:S laser (700-1000nm, 1 W cw)
- Doubled Nd:YAG (532nm, 10 mJ per pulse)
- Ar ion laser (488nm, 5 W cw)
- TE-cooled GaAs photon-counting PMT (Hamamatsu 943-02) (160-930nm) and Several other PMT’s
- TE-cooled MCP intensified CCD (400-900nm)
- TE-cooled Andor Newton EMCCD
- 0.85m SPEX double-grating Raman spectrometer
- Acton grating spectrometers: 0.75m, 0.5m, 0.15m (2)
- Princeton Instruments back-illuminated, deep-depletion CCD
- New Focus External Cavity Tunable Diode Laser
- Several CW diode and HeNe lasers
Terahertz Detectors
- Liquid He cooled Si bolometer (thermal-limit, 100 ms response)
- Liquid He cooled InSb bolometer (200ns response)
- Fast Ga:Ge photoconductor (moderate-sensitivity, 1 ns response)
- Pyroelectric detectors (low-sensitivity, 1 ns response)
- Thomas Keating absolute calibrated detector
- TACIT detector (under development, currently <1ns response) Cryogenics
- 8 Tesla Oxford superconducting optical cryostat
- 8 Tesla Oxford spectromag split-coil superconducting magnet
- 12 Tesla Oxford spectromag split-coil superconducting magnet
- Several liquid helium flow cryostats with optical access
- Several liquid nitrogen cooled detector housings
Lab Locations
Free Electron Laser Facility (1300 series rooms): Access to the FEL is through Broida Hall room 1357, located at the east end of the Broida Hall first floor south-facing outdoor walkway.Telephone numbers:
- Dave Enyeart’s Office: 893-3390
- Nick Agladze’s Office: 893-7023
- Student Office: 893-4707
- THz source and Detector Lab (1380B): 893-4707
- VNA Lab (1380C): 893-2423
- HSG Lab (1380D): 893-8396
- High Field EPR Lab (1390A): 893-8396
THz spectroscopy lab: Broida Hall room 1217, access via FTIR lab. Includes clean bench and chemical hood.
Techniques
Our experimental expertise is in special techniques for the terahertz frequency range. These involve a combination of far-infrared, near-infrared, ultrafast, and RF techniques at low temperature.Terahertz pulse slicing
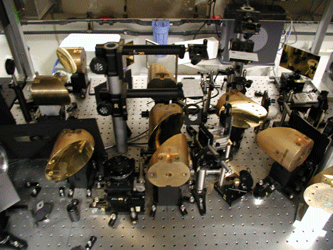
Terahertz photoconductivity
An intense terahertz pulse from the pulse slicer drives electrons in the device from localized to extended states. This results in a change in the conductivity in the RF frequency range. Coherent terahertz oscillations of electrons can be mapped out stroboscopically by measuring the photoconductivity vs. terahertz pulse length or delay.Optically-detected terahertz spectroscopy
In optically detected THz experiments, changes in the optical absorption, photoluminesence, or resonant scattering due to THz irradation are measured. We have used this technique to investigate collective dynamics of electrons in quantum wells, and recently, as a technique for reading out the quantum state of shallow donors in GaAs.Terahertz Time-Domain Spectroscopy
Broadband THz radiation with time duration on the order of a picosecond is generated in a semoconductor wafer by incident near-infrared (NIR) 150 fs laser pulses. The THz is created by either difference frequency mixing of the NIR in a semiconductor with a second-order susceptiblity nonlinearity, or by photo-created electrons accelerating across the surface depletion fields of a doped semiconductor.The THz is collimated, and sent collinearly with a probe beam from the femtosecond laser pulse through an electro-optic ZnTe crystal. The THz electric field rotates the polarization probe pulse via the Pockels effect. The rotation of the probe pulse is detected by a balanced optical bridge. The rotation is proportional to the magnitude of the THz electric field. By varying the time delay with of the probe pulse, the electric field of the THz can be measured. The THz spectrum is found by Fourier transforming the electric field and taking the square modulus.
THz transmission or reflection can be measured by placing a sample in the path of the terahertz and comparing the measured spectrum with a reference spectrum taken with no sample.
Terahertz-optical mixing spectroscopy
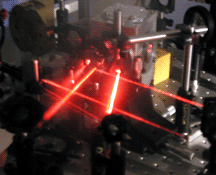
Fourier-Transform Infrared Spectroscopy (FTIR)
The FTIR is a Michelson interferometer used to measure infrared absorption of a sample. Our Bruker IFS 66v can be used in the near, mid and far IR, providing absorption spectra over an extremely wide spectral range (1-500um), using a combination of alkakli-halide and mylar beam splitters and InSb, HgCdTe (MCT), and bolometer detectors for detection.The FTIR sends a collimated thermal source with a broad emmision spectrum through a sample and into a Michelson interferometer. The instrument sweeps the mirror in one arm of the interfermeter at a constant rate. An interferogram is produced by measuring the intensity at the output of the interferemeter as a function of time. For low-light measurements the interferogram can be built up by repeatedly stepping the mirror and collecting a single data point.
The frequency spectrum is obtained by taking the Fourier transform of the interferogram and subtracting it from a reference spectrum taken with no sample. Our FTIR is mainly used to measure FIR intraband absorption spectra of coupled quantum well and dots, but we can also use it to measure the thickness of wafers by measuring the spacing between Fabry-Perot fringes.
There are many tips and tricks on our particular machine, a Bruker IFS 66v.